
Our idea
The challenge is to generate energy in Venusian conditions: high temperature, high pressure, corrosive atmosphere. So far, chemical, nuclear, wind and solar systems have been considered, but we decided to use a novel biomimetic approach. We start from a paper by Efrati et al. (2016) entitled "Assembly of photo-bioelectrochemical cells using photosystem I-functionalized electrodes" published in Nature energy. This paper presents a prototype solar panel using bacterial photosystems (chlorophyll+associated proteins) for the transformation of light into electricity with high efficiency. Our proposal is to take advantage of the chemical systems of extremophile bacteria that live in high temperatures and pressure, associated with submarine volcanoes, to make a solar panel or a chemical module that takes advantage of the conditions of Venus.

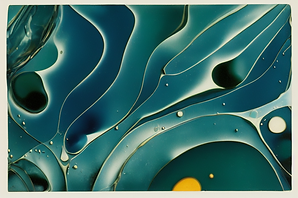.png)
Our project
With the aim of developing an energy storage system for Rovers or landing modules that will carry out activities on Venus, NASA and other associated space agencies have been searching
for different solutions to this dilemma. The most revisited are wind, solar, chemical, and even nuclear energy. Despite being the best studied, none offers sufficient guarantee to carry out a successful mission on this harsh planet.
.png)
With an average temperature of 464°C and a surface pressure of 92 bar, added to the strange chemical composition of its corrosive
atmosphere, it makes designing a mission to Venus a complete challenge. That's why, instead of trying to solve the problem from
scratch, we wanted to see if nature had already done it for us.
In our oceans, around submarine volcanoes, we find a possible answer, where bacterial photosystems play a fundamental role in solving the challenge and taking advantage of the Venusian atmosphere for energy generation.
Photosynthetic organisms capture a red photon and use its energy to excite an
electron in the chlorophyll, which will be consequently transferred and used to
promote chemical reactions where energy is stored. But a single chlorophyll
molecule cannot do everything alone, it requires other molecules that give it
structure and facilitate its action. Each complex of molecules with its chlorophyll
is called a photosystem.

The pioneering work of Efrati and collaborators in 2016 on a functional
prototype of artificial photosynthesis was our starting point. From this panel, we designed a power generation system capable not only of operating but also taking advantage of the atmospheric conditions of Venus.
For the resolution of this panel, we evaluated around 30 species extremophile bacteria, where one, in particular, stood out: GSB1, a bacteria capable of near-infrared photosynthesis using the glow of underwater magma as an energy source.

Our model is based on solar panels where the bacteriochlorophyll (PS1) and associated proteins from the GBS1 bacterium generate the energy needed to power the rover or lander.
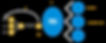
This consists of two subunits: on one side, the photosystem will be responsible for the capture of red and infrared and the delivery of electrons to the anode. On the other, for the regeneration of the photosystem, we have the oxidation of sulfur dioxide present in the Venusian atmosphere or the oxidation of sugars.
In this way, we can have enough energy efficiently and lightly.
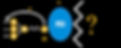
The system we propose is based on known and promising technology, although it is still experimental. Finding a substance that oxidizes sulfur dioxide under Venusian conditions is no small challenge, but it is a problem that the industry has experience with. And it is potentially a very light panel as it uses ITO and densely packed organic molecules.
09 Landis, G.A., Harrison, R., (2010). Batteries for Venus Suface Operation. Journal of propulsion and power Vol.26, 4, July-August 2010 Read
10 Jannasch, H. W., & Mottl, M. J. (1985). Geomicrobiology of deep-sea hydrothermal vents. Science, 229(4715), 717-725. Read
11 Dick, G. J. (2019). The microbiomes of deep-sea hydrothermal vents: distributed globally, shaped locally. Nature Reviews Microbiology, 17(5), 271-283. Read
12 Fujikura, K., Kojima, S., Tamaki, K., Maki, Y., Hunt, J., & Okutani, T. (1999). The deepest chemosynthesis-based community yet discovered from the hadal zone, 7326 m deep, in the Japan Trench. Marine Ecology Progress Series, 190, 17-26. Read
13 Beatty, J. T., Overmann, J., Lince, M. T., Manske, A. K., Lang, A. S., Blankenship, R. E., ... & Plumley, F. G. (2005). An obligately photosynthetic bacterial anaerobe from a deep-sea hydrothermal vent. Proceedings of the National Academy of Sciences, 102(26), 9306-9310. Read
14 Zavarzina, D. G., Sokolova, T. G., Tourova, T. P., Chernyh, N. A., Kostrikina, N. A., & Bonch-Osmolovskaya, E. A. (2007). Thermincola ferriacetica sp. nov., a new anaerobic, thermophilic, facultatively chemolithoautotrophic bacterium capable of dissimilatory Fe (III) reduction. Extremophiles, 11(1), 1-7. Read
15 Guezennec, J., Ortega-Morales, O., Raguenes, G., & Geesey, G. (1998). Bacterial colonization of artificial substrate in the vicinity of deep-sea hydrothermal vents. FEMS microbiology ecology, 26(2), 89-99. Read
16 Crespo-Medina, M. (2009). Diversity of chemosynthetic thiosulfate oxidizing bacteria from diffuse flow hydrothermal vents and their role in mercury detoxification. Rutgers The State University of New Jersey-New Brunswick. Read
17 Labrador, C. R., Hurtado, A. A. H., & Carrascal, J. I. S. (2018). Búsqueda de bacterias oxidadoras de azufre para su potencial uso en la producción de biogás de alta pureza. Revista de Investigación Agraria y Ambiental, 9(2), 295-304. Read
18 Van Dover, C. L. (2021). The ecology of deep-sea hydrothermal vents. In The Ecology of Deep-Sea Hydrothermal Vents. Princeton University Press. Read
19 Wood, B. E., Hess, P., Lustig‐Yaeger, J., Gallagher, B., Korwan, D., Rich, N., ... & Raouafi, N. E. (2022). Parker Solar Probe imaging of the night side of Venus. Geophysical Research Letters, 49(3), e2021GL096302.
01 Pérez Uria-Carril, E. (2009). Fotosíntesis: aspectos básicos. REDUCA (Biología) Vol. 2, Núm. 3 (2009)
02 Weliwatte, S. N., Grattieri, M., Shelley Mineteer, D. (2021). Rational design of artificial redox-meditating systems toward upgrading photobioelecrocatalysis. Photochemical & Photobiological Siences (2021) Read
03 Efrati, A., Chun-hua Lu., Michaeli, D., Nechushtai, R., Alsaoub, S., Schuhmann, W., Willner, I. (2016). Assembly of photo-biolectrochemical cells using photosystem I-functionalized electrodes. Nature energy Volume: 1, P: 1-8k Read
04 Kato, M ., Cardona, T., Rutherford, A. W., Reisner, E. (2012). Photoelectrochemical water oxidation with photosystem 2 integrated in a mesoporous indium-tin oxide electrode. Journal of the American Chemical Society, 134,8332-8335.
05 Brandon, E. J., Bugga, R., Grandidier, J., Hall, J. L., Schwartz, J. A., Limayne, S. (2020). power beaming for long life venus surface missions. Niac 2019 Phase 1
06 Surampudi, R., et al. (2017). Energy Storage Technologies for Future Planetary Science Missions. Nasa resources space apps challenge, Challenge: Exploring venus
07Bergh, C., Moroz., Taylor, F.W., Crisp, D., Bézard, B., Zasova, L.V. (2006). the composition of the atmosphere of venus below 100km altitude:an overview. Planetary and space science 54 (2006) Read
08 Zasova, L.V., Ignatiev, N., Khatuntsev, I., Linkin, V. (2007). Structure of the venus atmosphere. Planetary and space science 55 (2007) Read